“Kagome” metallic crystal adds new spin to electronics
A multinational team of researchers, co-led by a City University of Hong Kong (CityU) physicist, has found that a novel metallic crystal displays unusual electronic behaviour on its surface, thanks to the crystal’s unique atomic structure. Their findings open up the possibility of using this material to develop faster and smaller microelectronic devices.
The material that was studied is a recently discovered “kagome” metal compound that consists of three elements: gadolinium (Gd), vanadium (V), and tin (Sn). It is classed as a “1-6-6” material to indicate the ratio of the three metal elements present in the GdV6Sn6 crystal. The atoms are arranged in a complex but regular geometric pattern, resulting in extraordinary surface characteristics.
Normally, negatively charged electrons in atoms move about within discrete energy bands at different distances from the positively charged nuclei. However, on the surface of GdV6Sn6, top layers of exposed atoms are predicted to interact with each other and deform the topology, that is, shape and positioning, of the energy bands. In theory, this deformation could introduce a new and stable electronic property that, until now, has not been definitively detected in GdV6Sn6 or any other kagome metal.
First observation of unusual surface electronic behaviour in a kagome metal
“Our team unambiguously observed for the first time that a kagome metal can exhibit altered electronic energy-band structures of the type known as ‘topologically non-trivial Dirac surface states’,” says Dr Ma Junzhang, Assistant Professor in the Department of Physics at CityU. “Because of their intrinsic spin and charge, electrons create their own magnetic field and behave like tiny gyroscopes that have both rotation and an angled tilt that points in a certain direction. We demonstrated that in GdV6Sn6, the surface electrons become reordered or ‘spin-polarised’, and their tilts reorient themselves around a common axis that is perpendicular to the surface.”
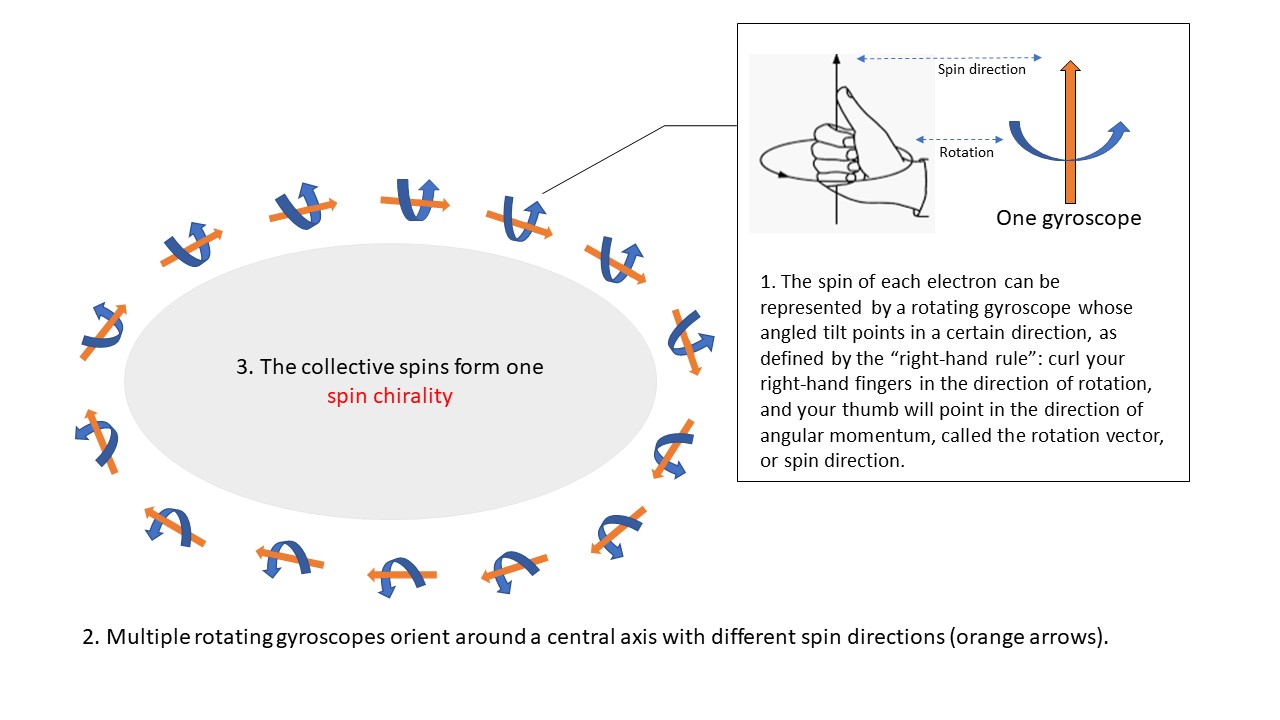
The ordered orientation of electrons around a shared axis is their “spin chirality”, which can be in either a clockwise or an anticlockwise direction (Fig. 1). More importantly, the research team was able to successfully reverse the spin chirality by performing a simple physical modification of the crystal surface. “Because we found that the spin chirality of the spin-polarised electrons is easily reversible, our material has great potential for application in next-generation transistors in the field of spintronics,” adds Dr Ma.
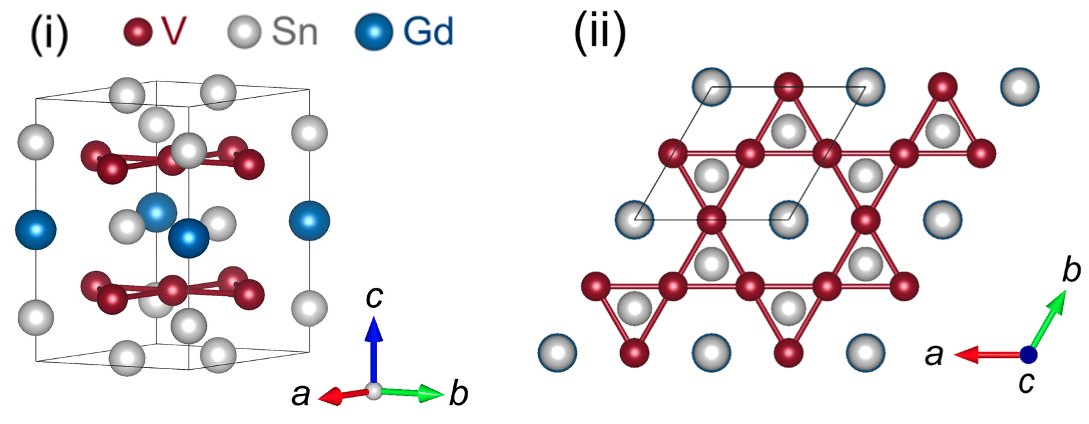
© Hu, Y. et al (Source: https://www.science.org/doi/10.1126/sciadv.add2024)
The study, published in Science Advances on 21 September 2022, was motivated by theoretical predictions of novel surface electronic band structures after considering special features of GdV6Sn6 kagome crystals. For example, layers of repeating V3Sn subunits are stacked between alternating layers of Sn and GdSn2 (Fig. 2(i)). Furthermore, multiple V3Sn subunits are arranged geometrically in a “kagome layer”, whose repeating pattern of six equilateral triangles surrounding a hexagon resembles the kagome lattice seen in Japanese bamboo basket weaving (Fig. 2(ii)). Finally, the V3Sn kagome layer is non-magnetic, whereas the GdSn2 layer is magnetic.
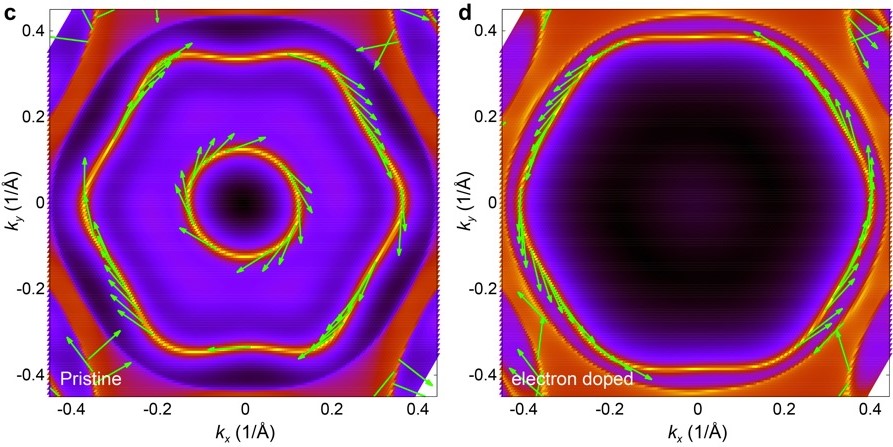
© Hu, Y. et al. (Source: https://www.science.org/doi/10.1126/sciadv.add2024)
First, the researchers made GdV6Sn6 crystals by heating Gd, V, and Sn metals and slowly cooling the product. Then, after confirming the chemical composition and structure by single-crystal X-ray diffraction, they cleaved a crystal through the stacked layers and analysed the exposed surface by angle-resolved photoemission spectroscopy, or ARPES. Results revealed that the cleaved surface indeed possessed reshaped energy band structures, and further analysis demonstrated clockwise spin character. Finally, the team showed that the surface energy bands could be warped drastically by coating the surface with potassium atoms, in a process known as electron doping. As a result, the electron spin chirality switched from clockwise to anticlockwise with increasing doping level (Fig. 3).
Potential applications in improving information transfer and beyond
The ability of researchers to deliberately reverse the spin chirality of surface electrons on the GdV6Sn6 crystal makes it a promising candidate material for numerous practical electronic applications.
“In the future, we might be able to apply a local voltage, or electrostatic ‘gate’, to directly manipulate or tune the electronic band structure and hence alternate the electron spin chirality on the surface of 1-6-6 kagome metals,” says Dr Ma. “Controlling the direction of spin-polarisation of electrons is an attractive alternative to traditional binary digital coding based on the presence or absence of electrical charge, which is relatively slow and can lead to problems such as device heating. Our technology could significantly increase efficiency in digital information transfer, with less heat generation, and could ultimately be exploited in quantum computing when coupled with superconductors.”
The first authors of the study are Dr Hu Yong from the Paul Scherrer Institute (PSI), Switzerland, and Dr Wu Xianxin from the Chinese Academy of Science, Beijing. The corresponding authors are Dr Hu, Dr Ma from CityU, and Professor Shi Ming from the PSI. Collaborators included Professor Xie Weiwei from Rutgers University, US, who provided the samples and Professor Andreas Schnyder from the Max Planck Institute, Germany.
In this work, Dr Ma was funded by CityU, the National Natural Science Foundation of China, and Guangdong Basic and Applied Basic Research Foundation. The other collaborators were supported by the Swiss National Science Foundation, Sino-Swiss Science and Technology Cooperation, National Natural Science Foundation of China, and US Department of Energy Basic Energy Sciences Program.