A new photoelectrochemical system: high solar-to-hydrogen conversion efficiency and low cost
Hydrogen is considered as future eco-friendly fuel due to its zero emissions in the production process. Using solar energy to generate hydrogen effectively through photoelectrochemistry has become a hot topic in the research and development sector in recent years. Researchers from the City University of Hong Kong (CityU) and King Abdullah University of Science and Technology have joined hands and successfully developed a novel photoelectrochemical system, which can greatly improve the solar-to-hydrogen conversion efficiency from 3% to nearly 9%. It is more stable, with stability lasting for over 150 hours, highest among its counterparts. And its cost is 50% lower.
Hydrogen can be produced from the photoelectrochemical reaction known as artificial photosynthesis. It is an environmental-friendly process, in which water is split into hydrogen and oxygen under sunlight with semiconductor as catalyst. However, due to technical limitations, the solar-to-hydrogen conversion efficiency is generally very low. Coupled with unstable performance and high cost, photoelectrochemical systems are therefore not widely used.
Semiconductors used in conventional systems are easily corroded
Professor He Jr-Hau, an expert in optoelectronic engineering from CityU’s Department of Materials Science and Engineering, pointed out that among the photoelectrochemical materials used for water splitting, III-V semiconductors, especially InGaP (Indium Gallium Phosphide) and GaAs (Gallium Arsenide) have attracted the most attention. These two compounds have excellent photophysical properties but poor photoelectrochemical stability. “III-V semiconductors undergo severe corrosion during the water splitting process due to their chemical instability. This will lead to catastrophic device failure,” he explained.
Professor He elaborated that almost all photoelectrochemical systems using silicon and III-V semiconductors possess the classical monofacial device configuration, in which all the major device components, including the light absorber, surface protection layer, and electrocatalysts, are integrated on only one side of the device, whereas the other side is used as a carrier collector to complete a circuit. This kind of configuration creates problems.
He explained that since the protection layer and electrocatalyst are typically opaque, putting them on top of the light-absorbing layer would hinder light absorption and reduce photocurrents during the water splitting process. Moreover, a portion of photons would be lost when they pass through the GaAs substrate in the conventional device.
Rearrange the light-absorbing and the electrocatalyst layers
To tackle the above shortcomings, Professor He and his research team have spent more than 3 years to design a new photoelectrochemical system. The new system not only resolved the light-blocking issue of the surface protection layer and the catalysts, but also reduced cost and improved system stability. Moreover, solar-to-hydrogen conversion efficiency is greatly improved.
Their research findings were published in the scientific journal Nature Communications, titled “An efficient and stable photoelectrochemical system with 9% solar-to-hydrogen conversion efficiency via InGaP/GaAs double junction”.
The uniqueness of their design lies in the repositioning the light-absorbing layer, catalyst, and protection layer. The catalyst layer that is usually placed as the top layer in conventional systems, is now placed as the bottom layer in the new system. This can help to reflect any unabsorbed long-wavelength photons back, enhancing their absorption. The new design also incorporates an anti-reflection layer to maximize the light harvesting capacity.
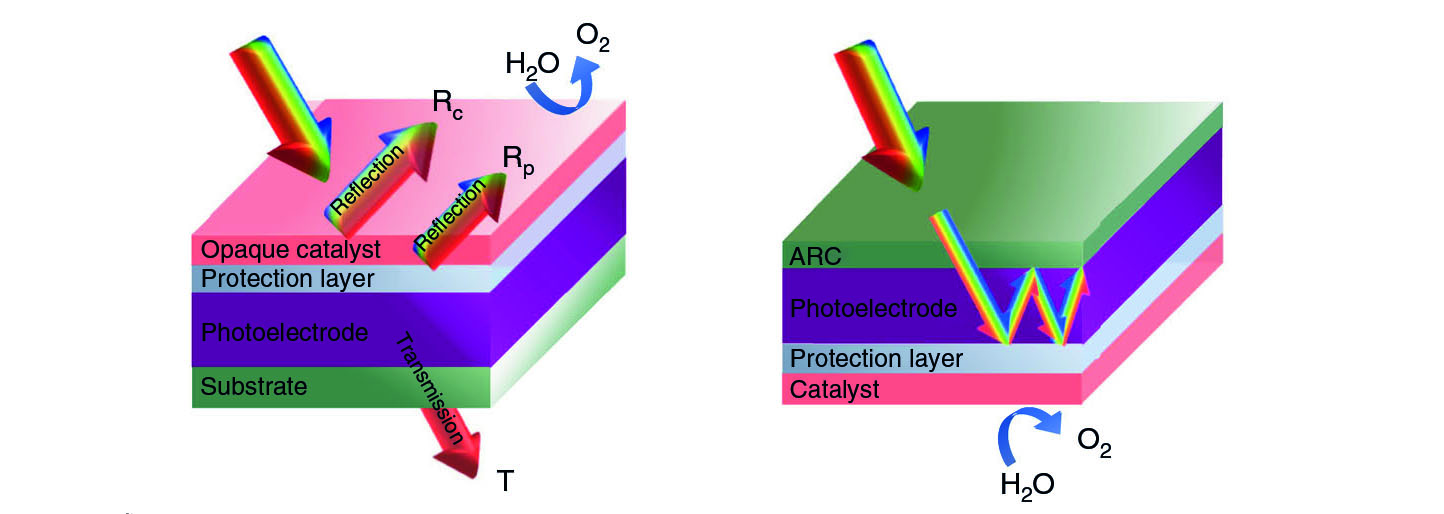
The experimental results showed that the new system has improved the solar-to-hydrogen conversion efficiency from 3% to nearly 9% under alkaline electrolyte, with photoelectrochemical stability of over 150 hours, the highest for any III-V system so far. In contrast, most of the similar systems would fail in a few hours or even in minutes.
Epitaxial lift-off method allows costly substrates to be reused
GaAs substrates that used to grow semiconductor layers are very expensive and generally account for 76% of the whole system cost. To reduce the cost, the research team deployed the epitaxial lift-off (ELO) method. Epitaxy is a process used to grow a thin crystalline layer on a crystalline substrate. For example, a light-absorbing III-V epitaxial layer can be grown on a GaAs substrate.
By applying the ELO method, the team is able to release and transfer the grown III-V semiconductor layer from the GaAs substrate to a nickel (Ni) substrate, and then carried out the photoelectrochemical reaction splitting water into oxygen and hydrogen. The original GaAs substrate could be reused to grow a new semiconductor epitaxial layer again. The new layer would then be released and transferred. By doing so, the cost is greatly reduced.
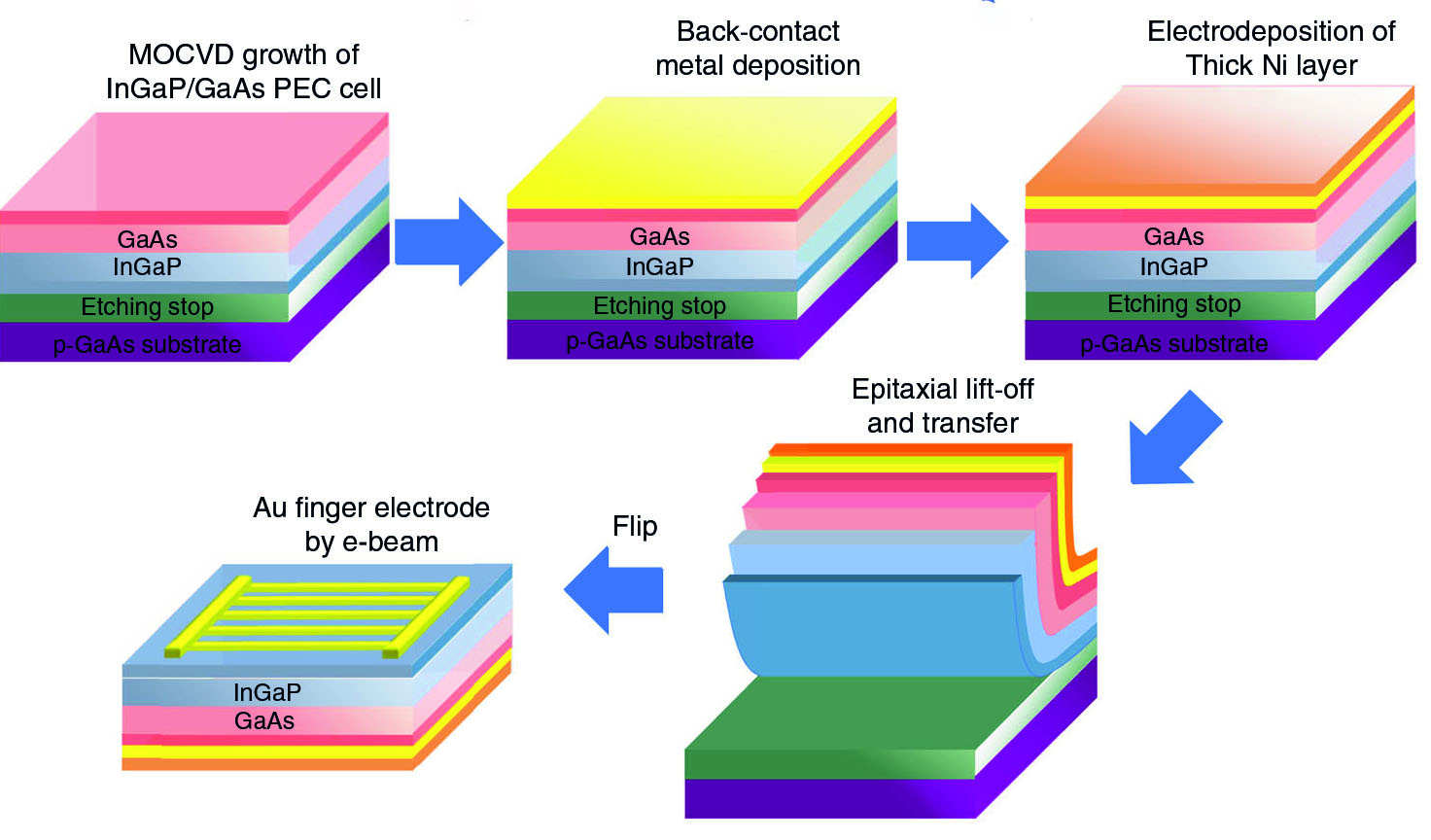
“Theoretically speaking, the released layer can be transferred to any surface through the ELO method. And by reusing GaAs substrates, the cost can be reduced by more than 50%,” said Professor He. He pointed out there are other advantages of using ELO method: multiple options for the surface protection materials, earth-abundant electrocatalyst which is generally opaque can be used without the concern of light blocking, and photon absorption can be maximized.
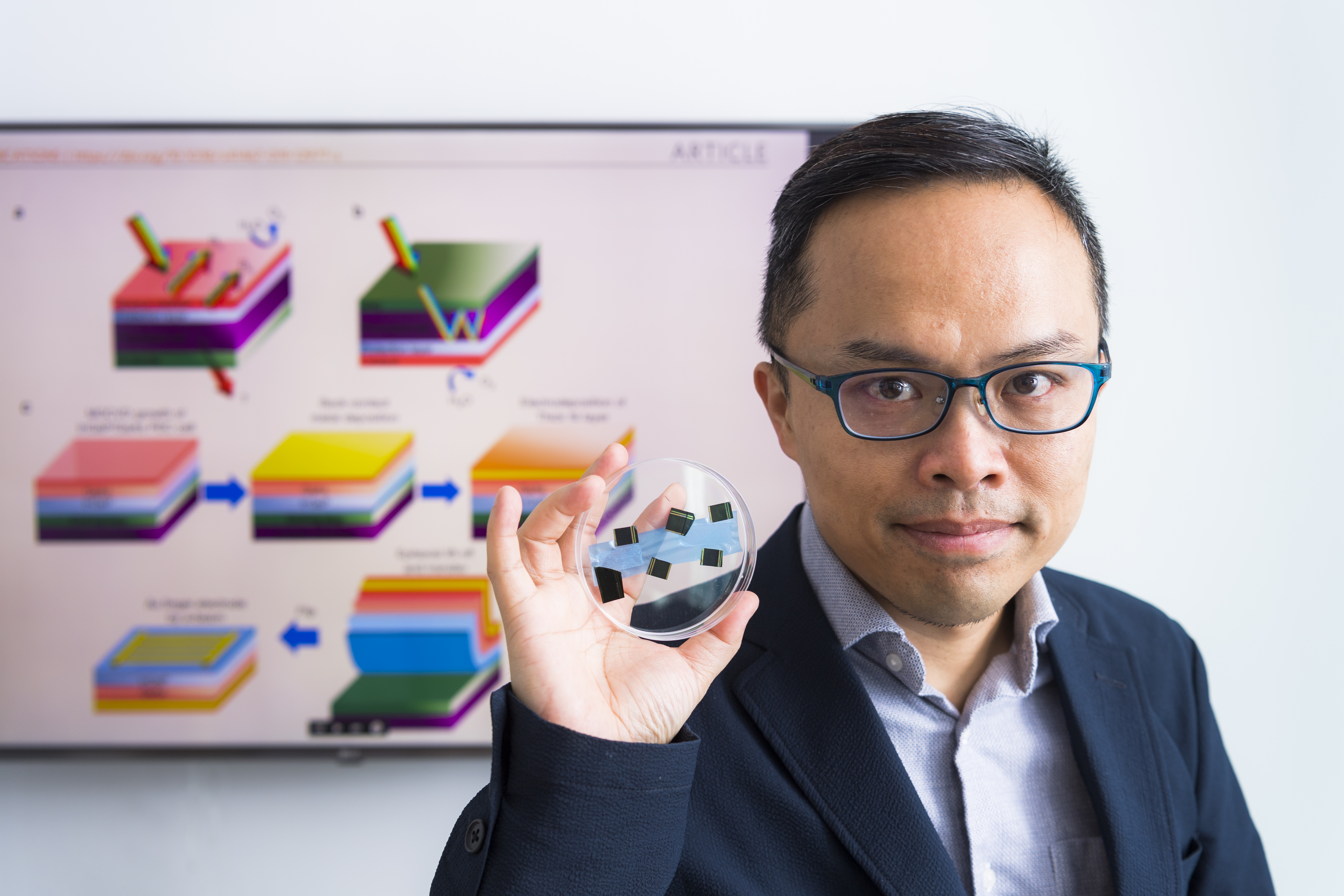
Enabled by the unique ELO and transfer method, both the front and back sides of the photoelectrochemical device can be fully utilized. Therefore, the team was able to construct a fully-integrated, unassisted-wireless “artificial leaf” system, with solar-to-hydrogen conversion efficiency of nearly 6%. “Unassisted-wireless” means that water can be split into oxygen and hydrogen without applying additional energy. “A maximum theoretical efficiency of 16% can be achieved with our new system. This is higher than the requirement of 10% set by the U.S. Department of Energy for commercialization,” said Professor He.
According to the data collected by the team, the hydrogen and oxygen gas production rates were stable throughout the monitoring period, indicating the device’s excellent stability without corrosion over time. Moreover, the released and transferred semiconductor layer is flexible. Researchers discovered that the layer can even be bent with radius of curvature of 2 mm, without compromising its water splitting function. Thus the device can be integrated onto flexible electronic modules.
The unassisted-wireless “artificial leaf” system is verified with the solar-to-hydrogen conversion efficiency of nearly 6%.
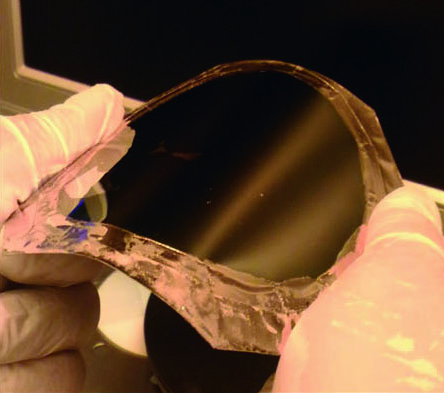
Professor He is the corresponding author of the paper. First authors are Dr. Purushothaman Varadhan, now a postdoctoral fellow, Dutch Institute for Fundamental Energy Research, Netherlands and Dr. Hui-Chun Fu, now a postdoctoral fellow at University of Wisconsin-Madison, USA. Professor He added that their team is building a larger scale prototype at CityU to study the feasibility of mass production of the system. The team has filed the technology for a patent application in the USA and mainland China.
The research was supported by King Abdullah University of Science and Technology.
DOI number: 10.1038/s41467-019-12977-x